Role of SPS temperature and holding time on the properties of Ti3AlC2-doped TiAl composites
- 1 Semiconductors Department, Materials and Energy Research Center (MERC), Karaj, Iran
- 2 Ceramics Department, Materials and Energy Research Center (MERC), Karaj, Iran
- 3 Faculty of Mechanical, Process and Energy Engineering, TU Bergakademie, Freiberg, Germany
Abstract
In order to study the effects of sintering conditions on the properties of TiAl-based materials, two different compositions (TiAl-15 wt% Ti3AlC2 and TiAl-25 wt% Ti3AlC2) were chosen and manufactured by spark plasma sintering at 900 °C/7 min and 1000 °C/15 min. The results showed that increasing the MAX phase content had a positive effect on the relative density and mechanical properties, but simultaneous increasing the temperature and holding time is more effective in the improvement of properties. For TiAl-15 wt% Ti3AlC2 sample, the relative density, Vickers hardness, fracture toughness, and bending strength increased from 92.3%, 3.6 GPa, 10.9 MPa.m1/2, and 206 MPa to 95.2%, 4.5 GPa, 12.0 MPa.m1/2, and 336 MPa, respectively, as the sintering temperature and holding time increased from 900 °C/7 min to 1000 °C/15 min. In the case of the TiAl-25 wt% Ti3AlC2 sample, increasing the sintering temperature and holding time from 900 °C/7 min to 1000 °C/15 min led to the improvement of relative density, Vickers hardness, fracture toughness, and bending strength from 92.8%, 4.1 GPa, 11.2 MPa.m1/2, and 270 MPa to 97.5%, 4.6 GPa, 11.8 MPa.m1/2, and 340 MPa, respectively.
Downloads
References
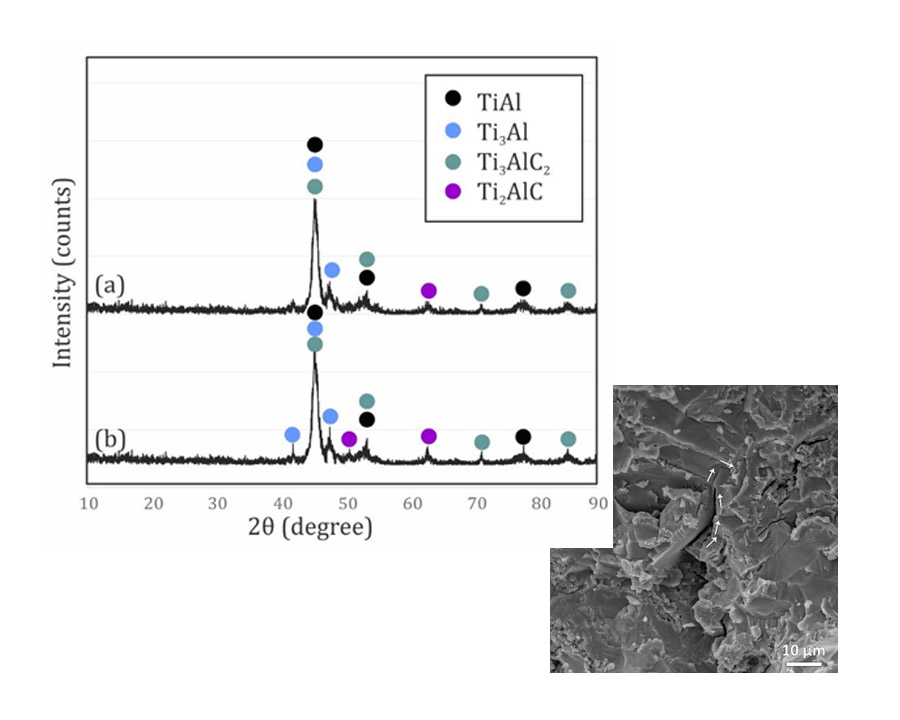
Copyright (c) 2022 Maryam Akhlaghi, Esmaeil Salahi, Seyed Ali Tayebifard, Gert Schmidt

This work is licensed under a Creative Commons Attribution 4.0 International License.
Copyright
Authors are the copyright holders of their published papers in Synthesis and Sintering, which are simultaneously licensed under a Creative Commons Attribution 4.0 International License. The full details of the license are available at https://creativecommons.org/licenses/by/4.0/.
All papers published open access will be immediately and permanently free for everyone to read, download, copy, distribute, print, search, link to the full-text of papers, crawl them for indexing, pass them as data to software, or use them for any other lawful purpose without any registration obstacles or subscription fees.