Role of Ti3AlC2 MAX phase on characteristics of in-situ synthesized TiAl intermetallics. Part II: phase evolution
- 1 Semiconductors Department, Materials and Energy Research Center (MERC), Karaj, Iran
- 2 Ceramics Department, Materials and Energy Research Center (MERC), Karaj, Iran
- 3 Faculty of Mechanical, Process and Energy Engineering, TU Bergakademie, Freiberg, Germany
Abstract
In this research, the 2nd part of a series of papers on the processing and characterization of TiAl–Ti3AlC2 composites, the phase evolution during the manufacturing process was investigated by X-ray diffraction (XRD) analysis and Rietveld refinement method. Metallic Ti and Al powders with different amounts of previously-synthesized Ti3AlC2 additives (10, 15, 20, 25, and 30 wt%) were ball-milled and densified by spark plasma sintering (SPS) under 40 MPa for 7 min at 900 °C. Before the sintering process, XRD test verified that the powder mixtures contained metallic Ti and Al as well as Ti3AlC2 and TiC (lateral phase synthesized with Ti3AlC2) phases. In the sintered composites, the in-situ synthesis of TiAl and Ti3Al intermetallics as well as the presence of Ti3AlC2 and the formation and Ti2AlC MAX phases were disclosed. The weight percentage of each phase in the final composition of the samples and the crystallite size of different phases were calculated by the Rietveld refinement method based on the XRD patterns. The size of Ti3AlC2 crystallites in sintered samples was compared with the crystallite size of synthesized Ti3AlC2 powder.
Downloads
References
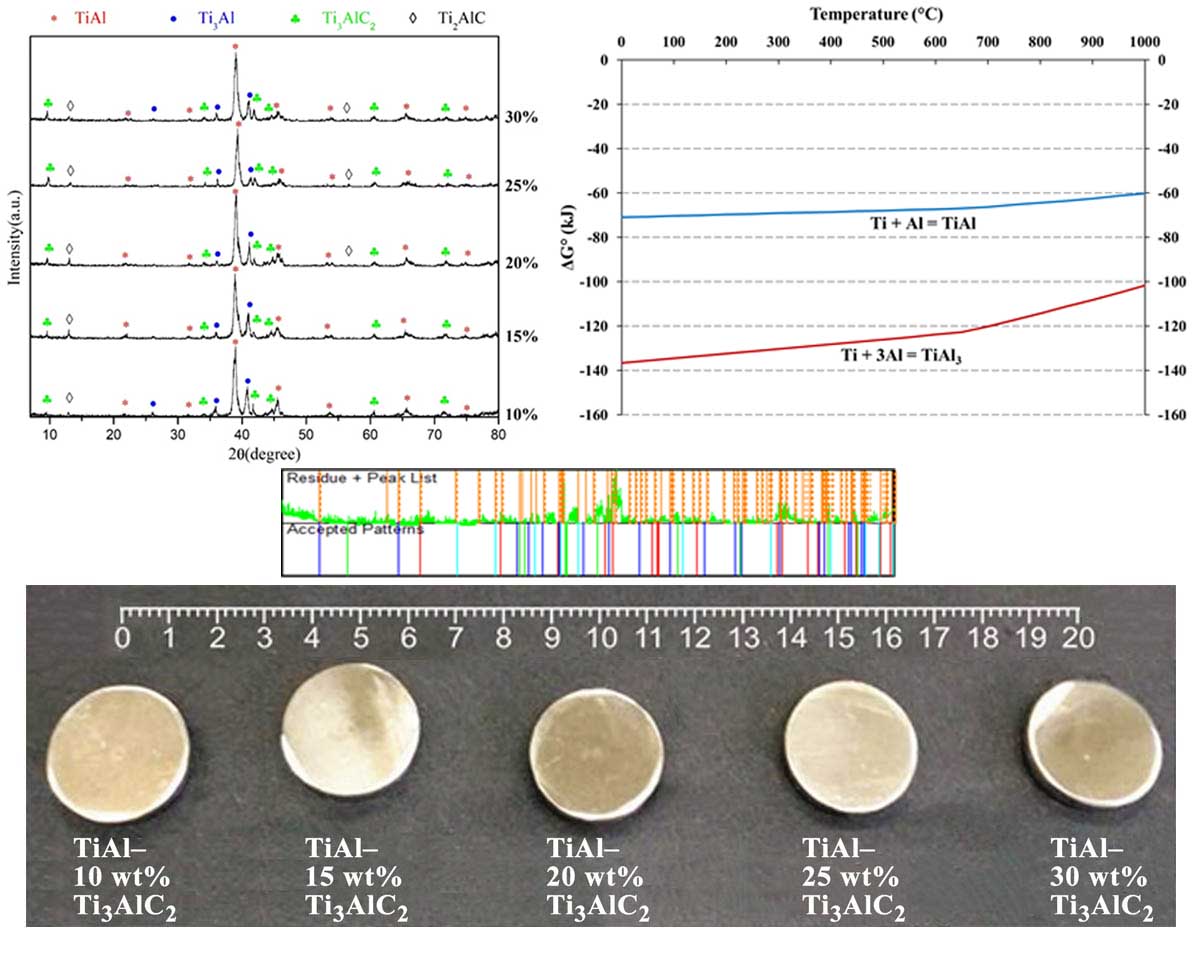
Copyright (c) 2021 Maryam Akhlaghi, Esmaeil Salahi, Seyed Ali Tayebifard, Gert Schmidt

This work is licensed under a Creative Commons Attribution 4.0 International License.
Copyright
Authors are the copyright holders of their published papers in Synthesis and Sintering, which are simultaneously licensed under a Creative Commons Attribution 4.0 International License. The full details of the license are available at https://creativecommons.org/licenses/by/4.0/.
All papers published open access will be immediately and permanently free for everyone to read, download, copy, distribute, print, search, link to the full-text of papers, crawl them for indexing, pass them as data to software, or use them for any other lawful purpose without any registration obstacles or subscription fees.