Effects of clay and fireclay addition on the properties of magnesia–forsterite–spinel refractories synthesized at different firing temperatures
- 1 Ceramics Department, Materials and Energy Research Center (MERC), Karaj, Iran
- 2 Department of Mechanical and Manufacturing Engineering, Faculty of Engineering and Built Environment, Universiti Kebangsaan Malaysia, Bangi, 43600, Selangor, Malaysia
Abstract
This study investigates the effects of adding clay and fireclay on the physical and mechanical properties of magnesia-based refractories such as contraction, bending strength, bulk density, and apparent porosity. Domestic raw materials were used for the preparation of samples fired at 1350, 1450, and 1550 °C for 2 h. Adding clay exhibited no significant effect on the density and porosity, whereas adding fireclay had a remarkable influence on the shrinkage. Nevertheless, the effects of clay and fireclay on the strength of magnesia were unnoticeable. X-ray diffraction results showed that, after firing, the main phase compositions of the samples with clay addition were periclase and forsterite. Adding fireclay led to the synthesis of magnesite spinel, which can be attributed to the high alumina content. Based on scanning electron microscopy, no liquid phase was formed indicating that the sintering was a solid-state evolution with the synthesis of forsterite.
Downloads
References
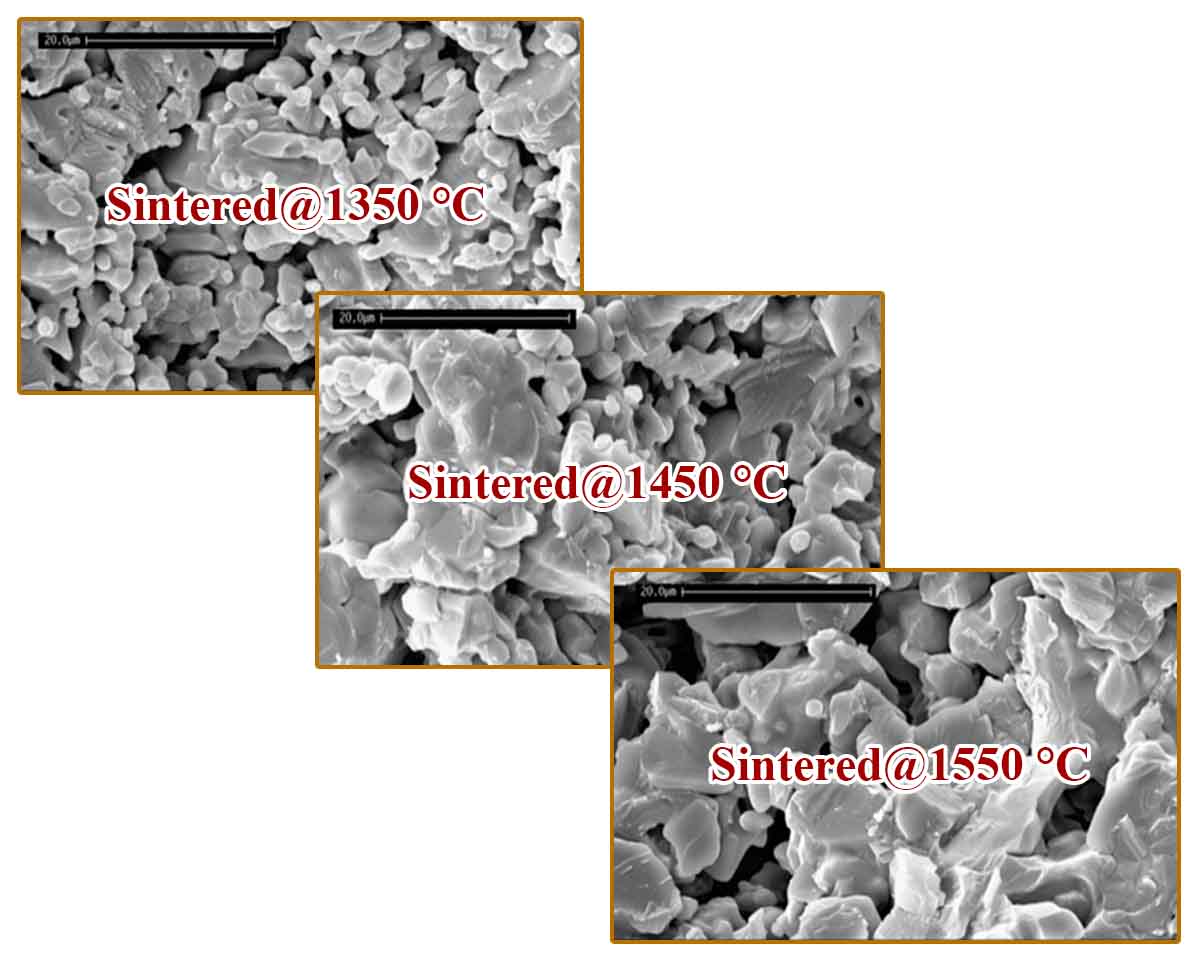
Copyright (c) 2022 Esmaeil Salahi, M. Ghaffari, A. Faeghinia, A. Rajabi

This work is licensed under a Creative Commons Attribution 4.0 International License.
Copyright
Authors are the copyright holders of their published papers in Synthesis and Sintering, which are simultaneously licensed under a Creative Commons Attribution 4.0 International License. The full details of the license are available at https://creativecommons.org/licenses/by/4.0/.
All papers published open access will be immediately and permanently free for everyone to read, download, copy, distribute, print, search, link to the full-text of papers, crawl them for indexing, pass them as data to software, or use them for any other lawful purpose without any registration obstacles or subscription fees.