Role of Ti3AlC2 MAX phase on characteristics of in-situ synthesized TiAl intermetallics. Part I: sintering and densification
- 1 Central Reference Laboratory, Iran University of Science and Technology, Narmak, Tehran, 16844, Iran
- 2 Ceramics Department, Materials and Energy Research Center (MERC), Karaj, Iran
- 3 Semiconductors Department, Materials and Energy Research Center (MERC), Karaj, Iran
- 4 Faculty of Mechanical, Process and Energy Engineering, TU Bergakademie, Freiberg, Germany
Abstract
Five TiAl–Ti3AlC2 composite samples containing (10, 15, 20, 25 and 30 wt% Ti3AlC2 MAX phase) were prepared by spark plasma sintering technique at 900 °C for 7 min under 40 MPa. For this purpose, metallic titanium and aluminum powders (aiming at the in-situ formation of the TiAl matrix phase) were ball-milled with predetermined contents of Ti3AlC2 MAX phase, which already was synthesized using the same metallic powders as well as graphite flakes. Displacement-time-temperature variations during the heating and sintering steps, displacement rate versus temperature, displacement rate versus time, and densification behavior were studied. Two sharp changes were detected in the diagrams: the first one, ~16 min after the start of the heating process due to the melting of Al, and the second one, after ~35 min because of the sintering progression and the applied final pressure. The highest relative densities were measured for the samples doped with 20 and 25 wt% Ti3AlC2 additives. More Ti3AlC2 addition resulted in decreased relative density because of the agglomeration of MAX phase particles.
Downloads
References
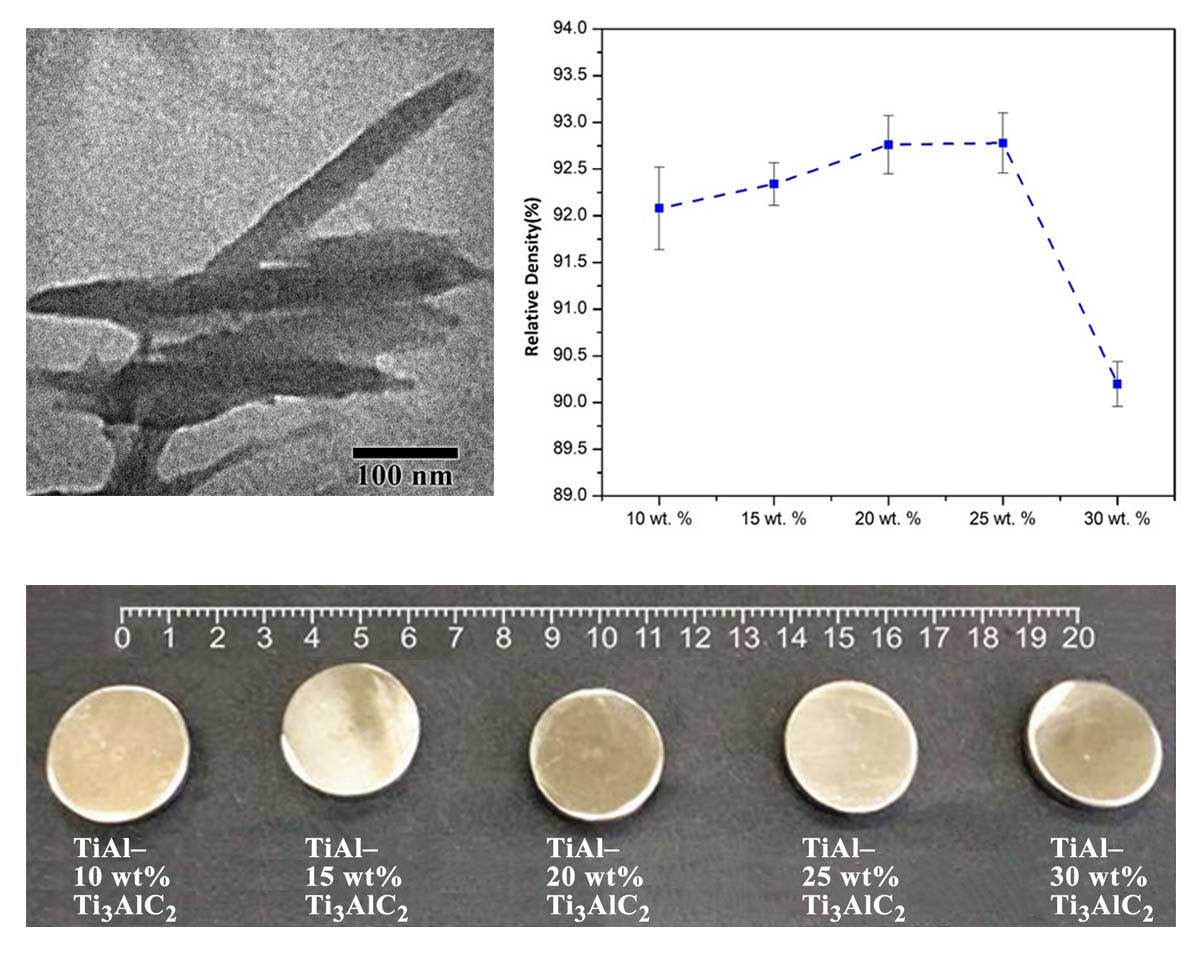
Copyright (c) 2021 Maryam Akhlaghi, Esmaeil Salahi, Seyed Ali Tayebifard, Gert Schmidt

This work is licensed under a Creative Commons Attribution 4.0 International License.
Copyright
Authors are the copyright holders of their published papers in Synthesis and Sintering, which are simultaneously licensed under a Creative Commons Attribution 4.0 International License. The full details of the license are available at https://creativecommons.org/licenses/by/4.0/.
All papers published open access will be immediately and permanently free for everyone to read, download, copy, distribute, print, search, link to the full-text of papers, crawl them for indexing, pass them as data to software, or use them for any other lawful purpose without any registration obstacles or subscription fees.