Hydrogen evolution via noble metals based photocatalysts: A review
- 1 Department of Chemical Engineering, Arak Branch, Islamic Azad University, Arak, Iran
- 2 School of Physics and Materials Science, Shoolini University of Biotechnology and Management Sciences, Solan 173212, India
- 3 Department of Chemical Engineering, Universiti Teknologi PETRONAS, Bandar Seri Iskandar, 32610, Perak, Malaysia
- 4 Department of Chemistry, University of Botswana, Notwane Rd, P/bag UB 00704, Gaborone, Botswana
- 5 College of Energy Source and Mechanical Engineering, Shanghai University of Electric Power, Shanghai 200090, People’s Republic of China
- 6 Luxi Polymetal Co. Ltd., Abuja, Nigeria
Abstract
In recent decades, the use of photocatalysts in the evolution of hydrogen (H2) has received much attention. However, the use of the well-known titanium oxide and another photocatalyst as a base for noble metals is limited due to their major weakness in electron-hole pair separation. The use of cocatalysts can be a good way to overcome this problem and provide better performance for the evolution of hydrogen. In this review, suitable high-efficiency cocatalysts for solar hydrogen production have been thoroughly reviewed. New strategies and solutions were examined in terms of increasing the recombination of charge carriers, designing reactive sites, and enhancing the wavelengths of light absorption. Several new types of cocatalysts based on semiconductors in noble groups and dual metals have been evaluated. It is expected that these photocatalysts will be able to reduce the activation energy of reaction and charge separation. In this regard, the existing views and challenges in the field of photocatalysts are presented. The characteristics of monoatomic photocatalysts are reviewed in this manuscript and the latest advances in this field are summarized. Further, the future trends and upcoming research are also briefly discussed. Finally, this review presents noble metal-based photocatalysts for providing suitable photocatalysts on a larger scale and improving their applicability.
Downloads
References
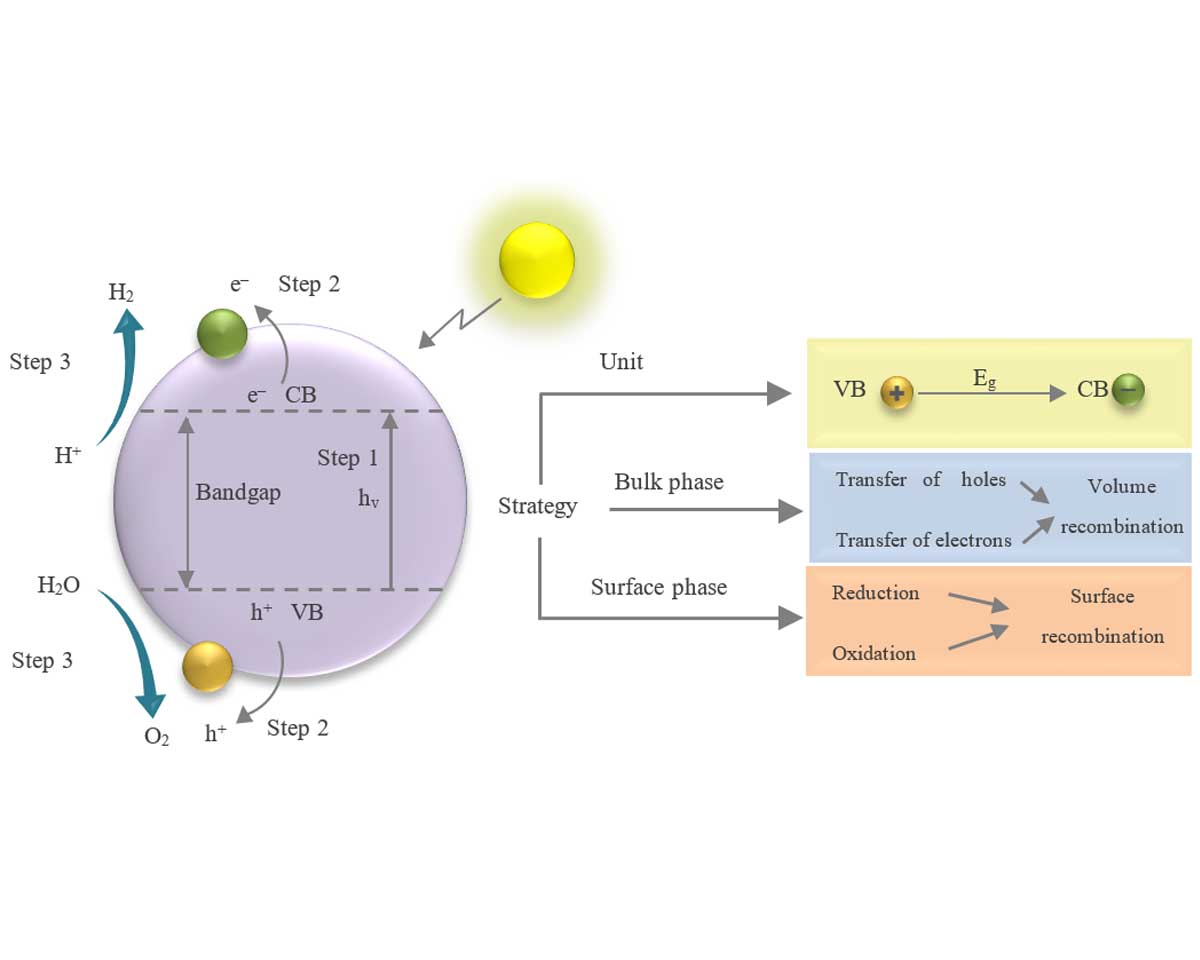
Copyright (c) 2021 Asieh Akhoondi, Ankush Sharma, Dinesh Pathak, Mohammad Yusuf, Taye B. Demissie, Rui-tang Guo, Adnan Ali

This work is licensed under a Creative Commons Attribution 4.0 International License.
Copyright
Authors are the copyright holders of their published papers in Synthesis and Sintering, which are simultaneously licensed under a Creative Commons Attribution 4.0 International License. The full details of the license are available at https://creativecommons.org/licenses/by/4.0/.
All papers published open access will be immediately and permanently free for everyone to read, download, copy, distribute, print, search, link to the full-text of papers, crawl them for indexing, pass them as data to software, or use them for any other lawful purpose without any registration obstacles or subscription fees.