Direct catalytic production of dimethyl ether from CO and CO2: A review
- 1 Department of Chemical Engineering, Arak Branch, Islamic Azad University, Arak, Iran
- 2 School of Chemistry and Chemical Engineering, Queen’s University Belfast, Belfast BT9 5AG, Northern Ireland, UK
- 3 Institute of Condensed Matter and Nanosciences (IMCN), Université catholique de Louvain (UCLouvain), Place Louis Pasteur 1, 1348, Louvain-la-Neuve, Belgium
Abstract
Dimethyl ether (DME) is a synthetically produced alternative fuel to diesel-based fuel and could be used in ignition diesel engines due to increasing energy demand. DME is considered extremely clean transportation and green fuel because it has a high cetane number (around 60), low boiling point (-25 °C), and high oxygen amount (35 wt%) which allow fast evaporation and higher combustion quality (smoke-free operation and 90% fewer NOx emissions) than other alternative CO2-based fuels. DME can be synthesized from various routes such as coal, petroleum, and bio-based material (i.e., biomass and bio-oil). Dimethyl ether can be produced from CO2 to prevent greenhouse gas emissions. This review aims to summarize recent progress in the field of innovative catalysts for the direct synthesis of dimethyl ether from syngas (CO+H2) and operating conditions. The problems of this process have been raised based on the yield and selectivity of dimethyl ether. However, regardless of how syngas is produced, the estimated total capital and operating costs in the industrial process depend on the type of reactor and the separation method.
Downloads
References
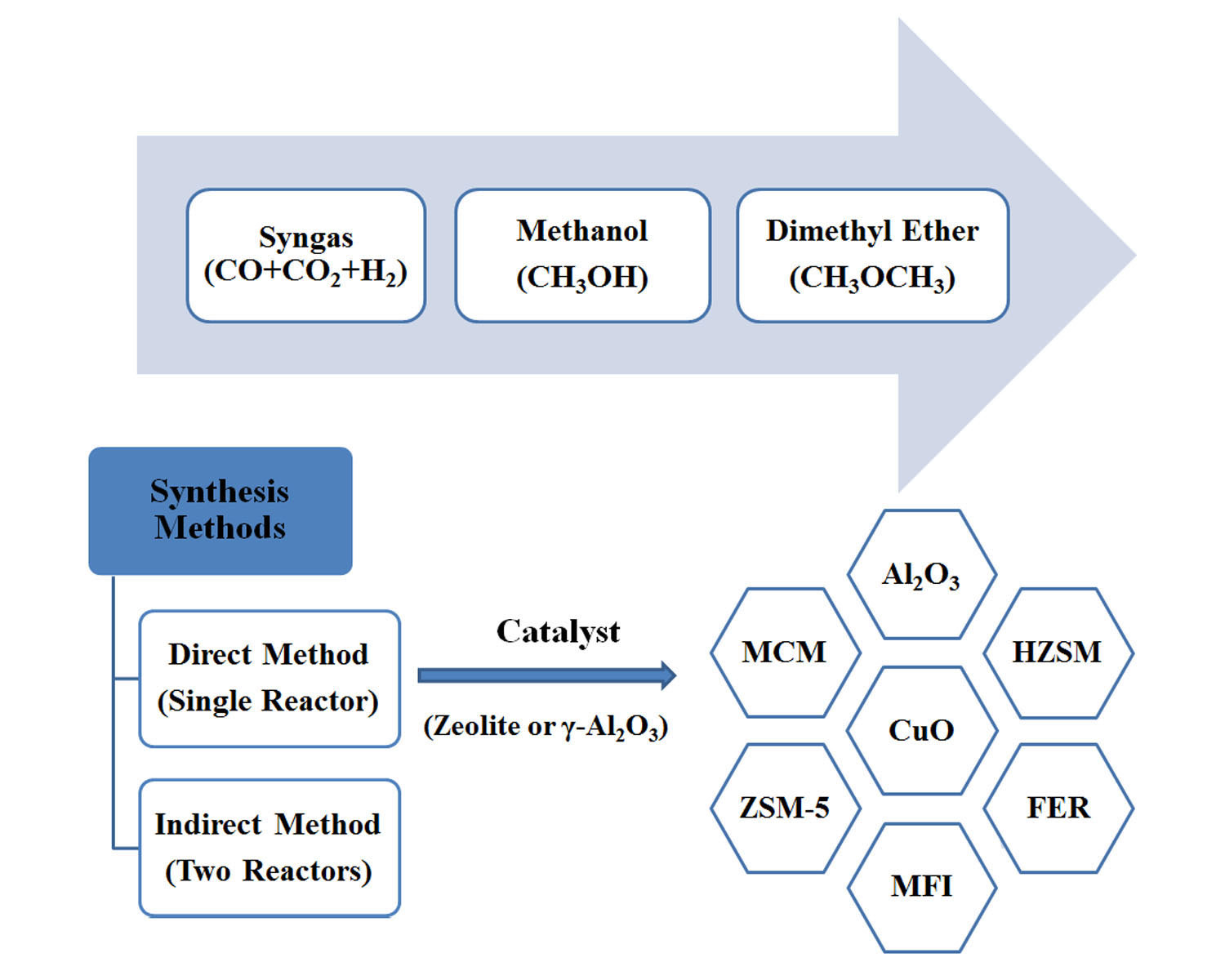
Copyright (c) 2021 Asieh Akhoondi, Ahmed I. Osman, Ali Alizadeh Eslami

This work is licensed under a Creative Commons Attribution 4.0 International License.
Copyright
Authors are the copyright holders of their published papers in Synthesis and Sintering, which are simultaneously licensed under a Creative Commons Attribution 4.0 International License. The full details of the license are available at https://creativecommons.org/licenses/by/4.0/.
All papers published open access will be immediately and permanently free for everyone to read, download, copy, distribute, print, search, link to the full-text of papers, crawl them for indexing, pass them as data to software, or use them for any other lawful purpose without any registration obstacles or subscription fees.