Advances in metal-based vanadate compound photocatalysts: synthesis, properties and applications
- 1 Department of Chemical Engineering, Arak Branch, Islamic Azad University, Arak, Iran
- 2 Institute for Nanotechnology and Water Sustainability (iNanoWS), Florida Campus, College of Science, Engineering and Technology, University of South Africa, Johannesburg 1709, South Africa
- 3 Department of Chemical Engineering, B. V. Raju Institute of Technology, Narsapur, Medak (Dist.), Telangana State, India
- 4 Institute for Nanotechnology and Water Sustainability (iNanoWS), Florida Campus, College of Science, Engineering and Technology, University of South Africa, Johannesburg 1709, South Africa
- 5 College of Rare Earths, Jiangxi University of Science and Technology, No.86, Hongqi Ave., Ganzhou, Jiangxi, 341000, PR China
Abstract
Among the ongoing research on photocatalysis under visible-light, it has been shown that doped or hybrid catalysts are more active than a single catalyst alone. However, problems including visible light absorption, a low quantity of energetic sites on surfaces, and rapid recombination of the photo-electron hole pair produced by light have prohibited photocatalysts from being used in a practical and widespread manner. To overcome these problems, synthesis of nanostructure hybrid catalyst using several methods has attracted much attention. Several procedures have been suggested for the preparation of photocatalysts with the desired structure and morphology. Preparation methods similar to partial modification may lead to diverse structures and qualities. In this regard, the development of efficient, low-cost photocatalysts and rapid synthesis is the most important issues that should be considered. This review discusses various methods and mechanisms that work with the modification of vanadium compounds as photocatalysts to progress their photocatalytic efficiency. In addition, the effects of synthesis temperature, solution pH and concentration on the photocatalytic performance are also described in detail.
Downloads
References
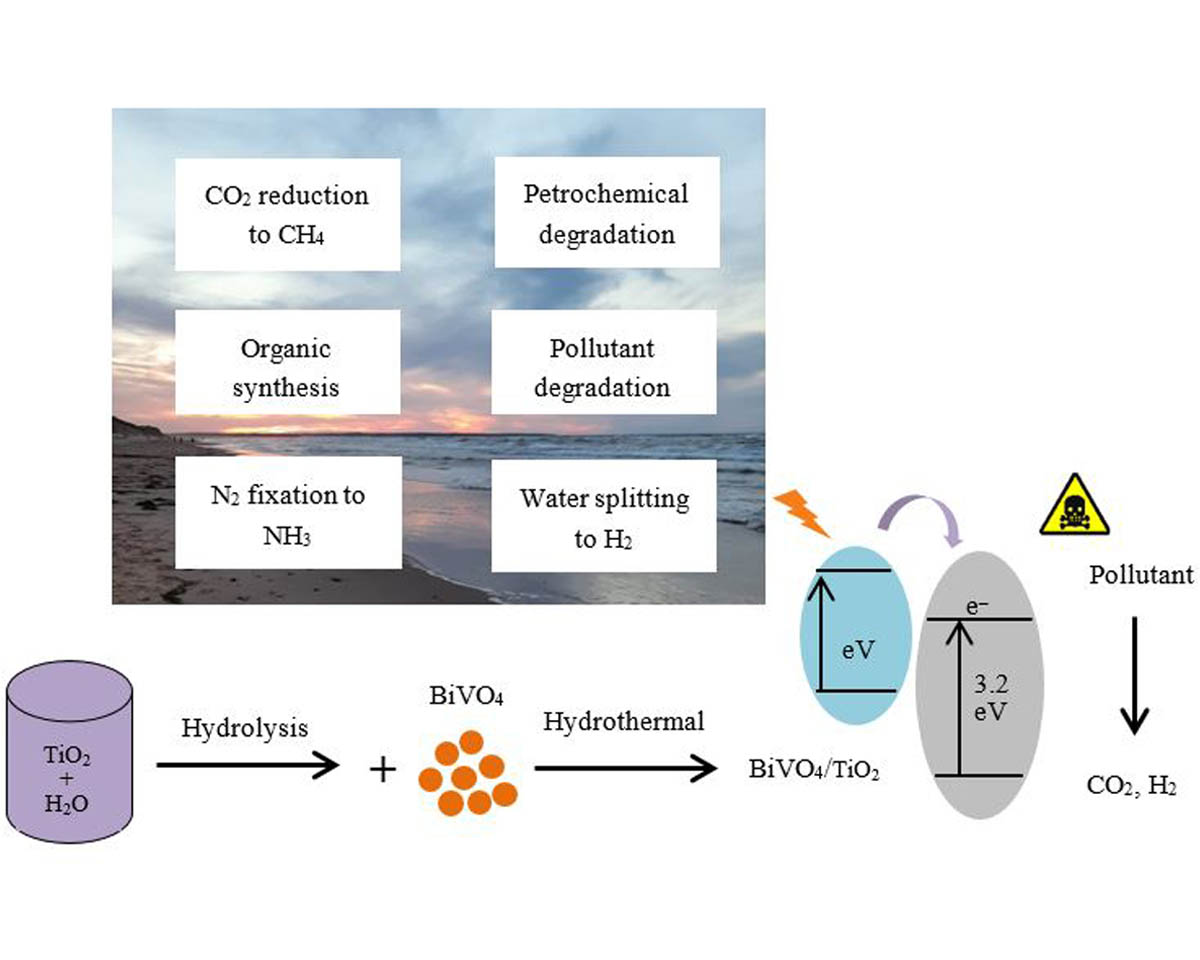
Copyright (c) 2021 Asieh Akhoondi, Usisipho Feleni, Bhaskar Bethi, Azeez Olayiwola Idris, Akbar Hojjati-Najafabadi

This work is licensed under a Creative Commons Attribution 4.0 International License.
Copyright
Authors are the copyright holders of their published papers in Synthesis and Sintering, which are simultaneously licensed under a Creative Commons Attribution 4.0 International License. The full details of the license are available at https://creativecommons.org/licenses/by/4.0/.
All papers published open access will be immediately and permanently free for everyone to read, download, copy, distribute, print, search, link to the full-text of papers, crawl them for indexing, pass them as data to software, or use them for any other lawful purpose without any registration obstacles or subscription fees.