New strategies in the preparation of binary g-C3N4/MXene composites for visible-light-driven photocatalytic applications
- 1 Department of Chemical Engineering, Arak Branch, Islamic Azad University, Arak, Iran
- 2 Nanomaterials Group, Department of Materials Engineering, Tarbiat Modares University, P.O. Box 14115-143, Tehran, Iran
- 3 Chemistry Department, Faculty of Science, Benha University, Benha, 13518, Egypt
- 4 Department of Chemical Engineering, Faculty of Engineering, Ferdowsi University of Mashhad, Mashhad, Iran
- 5 Chemical and Material Engineering Department, Esfarayen University of Technology, Esfarayen, Iran
- 6 Department of Petroleum Engineering, Universiti Teknologi PETRONAS, Bandar Seri Iskandar, Perak, 32610, Malaysia
Abstract
In recent years, g-C3N4@MXene photocatalysts have received much attention due to their special composition and excellent properties. MXenes consisting of transition metal carbides, nitrides, and carbonitrides derived from the MAX phase are used as cocatalysts or g-C3N4 (GCN) supporting composites in a variety of photocatalytic processes that accelerate the separation of charge carriers with their heterojunction structure. In addition to the high ability of g-C3N4@MXene nanocomposite to absorb light, it has high photocorrosion resistance in the processes of hydrogen evolution, wastewater treatment, nitrogen fixation, NO treatment, and oxidation and reduction photoreactions. In this review, the latest developments and new technologies for the manufacture and application of noble metal-free g-C3N4@MXene nanocomposite have been discussed and the future perspective has been drawn to deal with challenges related to energy and the environment.
Downloads
References
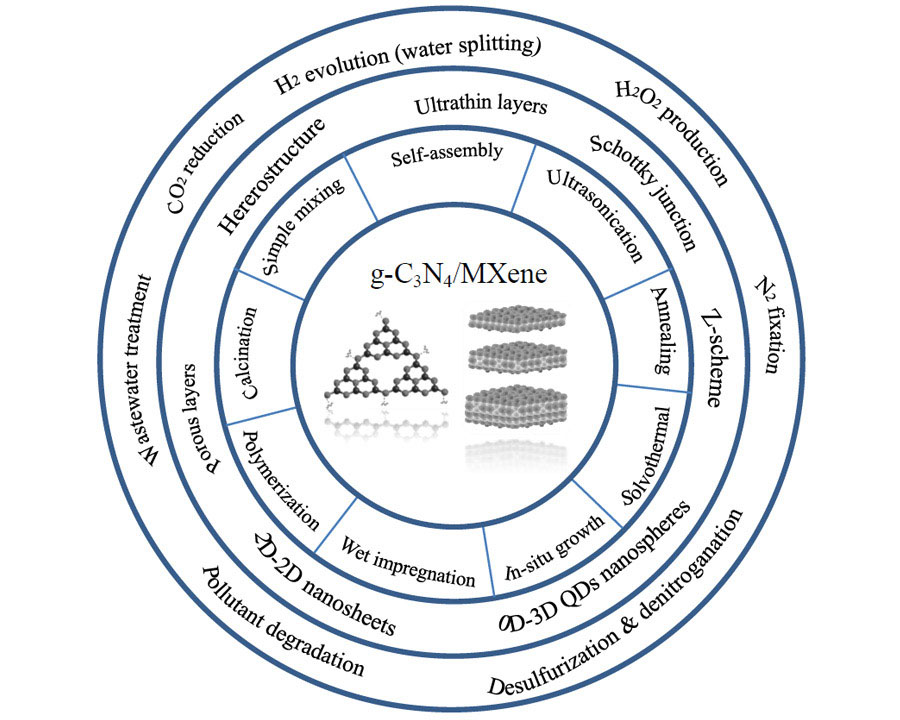
Copyright (c) 2022 Asieh Akhoondi, Mehrdad Mirzaei, Mostafa Y. Nassar, Zahra Sabaghian, Farshid Hatami, Mohammad Yusuf

This work is licensed under a Creative Commons Attribution 4.0 International License.
Copyright
Authors are the copyright holders of their published papers in Synthesis and Sintering, which are simultaneously licensed under a Creative Commons Attribution 4.0 International License. The full details of the license are available at https://creativecommons.org/licenses/by/4.0/.
All papers published open access will be immediately and permanently free for everyone to read, download, copy, distribute, print, search, link to the full-text of papers, crawl them for indexing, pass them as data to software, or use them for any other lawful purpose without any registration obstacles or subscription fees.