Recent advances in the synthesis of ZnO-based electrochemical sensors
- 1 Department of Chemical Engineering, Arak Branch, Islamic Azad University, Arak, Iran
- 2 Nanomaterials Research Group, Physics Division PINSTECH, Islamabad 44000, Pakistan
- 3 Henan Key Laboratory of Laser and Opto-electric Information Technology, School of Information Engineering, Zhengzhou University, Zhengzhou, Henan 450001, P. R. China
- 4 Materials Laboratory, Department of Chemistry, Mirpur University of Science and Technology (MUST), Mirpur, (AJK), Pakistan
- 5 Department of System Analysis and Management, Faculty of Economics, Empress Catherine II Saint Petersburg Mining University, Saint Petersburg, Russia
- 6 State Key Laboratory for Modification of Chemical Fibers and Polymer Materials, College of Materials Science and Engineering, Donghua University, 201620, Shanghai, P. R. China
- 7 Department of Computer Engineering, National University of Technology, Islamabad, 44000, Pakistan
Abstract
Until now, various composites based on zinc oxide (ZnO) have been investigated in electrochemical sensors. The physical and electrochemical properties of ZnO and its structure can improve the selectivity, sensitivity, and adaptability of nanocomposites. Therefore, the focus on the fabrication of cheap ZnO-based electrodes with affordable and easy transportability has increased. In addition, the electrochemical behavior is affected by the structure and morphology of the ZnO-based composite in detecting pollutants such as volatile organic compounds, heavy metals, and toxins. Furthermore, ZnO-based nanostructures are efficient in the fabrication of electrochemical sensors in the food industry, pharmaceutical analysis, and medical diagnostics. In this review, various techniques in the synthesis of ZnO-based electrodes and their effect on the particle size, shape, and morphology of compounds have been collected. Since the performance of chemical sensors has a direct relationship with the structure of the composite used in its electrode, it is necessary to discuss the new production methods, new concepts, strategies, and challenges. Additionally, new gains highlight recent developments and sensing of various analytes in the monitoring systems. These sensors have demonstrated a strong growth acceleration which could lead to the development of recent technologies. At last, an optimistic outlook is provided on the future of ZnO-based sensors and their challenges.
Downloads
References
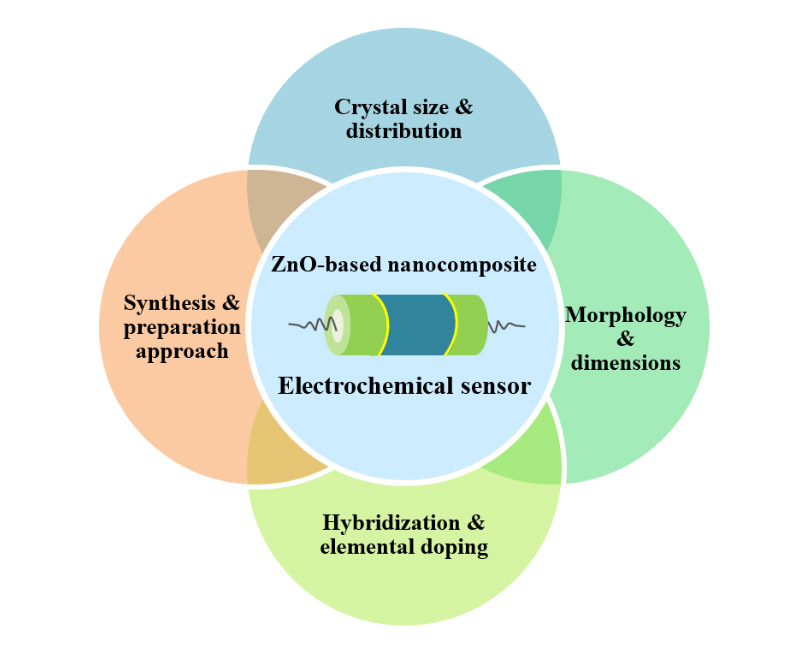
Copyright (c) 2023 Asieh Akhoondi, Mashkoor Ahmad, Muhammad Nawaz Sharif, Shahid Aziz, Hadi Davardoost, Qamar Wali, Faiza Jan Iftikhar

This work is licensed under a Creative Commons Attribution 4.0 International License.
Copyright
Authors are the copyright holders of their published papers in Synthesis and Sintering, which are simultaneously licensed under a Creative Commons Attribution 4.0 International License. The full details of the license are available at https://creativecommons.org/licenses/by/4.0/.
All papers published open access will be immediately and permanently free for everyone to read, download, copy, distribute, print, search, link to the full-text of papers, crawl them for indexing, pass them as data to software, or use them for any other lawful purpose without any registration obstacles or subscription fees.