Recent advances in synthesis of MXene-based electrodes for flexible all-solid-state supercapacitors
- 1 Department of Chemical Engineering, Arak Branch, Islamic Azad University, Arak, Iran
- 2 Chemistry Department, Faculty of Science, Benha University, Benha, 13518, Egypt
- 3 Advanced Functional Materials Research Group, Institut Teknologi Bandung, Bandung 40132, Indonesia
- 4 Division of Process Engineering, College of Science and Technology, Chadli Bendjedid University, 36000, Algeria
Abstract
Various energy storage sources have been developed so far, among which supercapacitors are more important for the forthcoming generations due to their small size and portability. Supercapacitors as good alternatives to batteries have recently attracted more attention because they have higher power and excellent charging-discharging rate which is considered as a challenging issue that limits the use of batteries. Supercapacitors also have other advantages over batteries, including higher reversibility and cycle life, lower maintenance costs, and safer electrode materials. MXenes have emerged as a new class of 2D composites in electrode materials for supercapacitors as low-cost and environment-friendly carbides and nitrides. MXenes are suitable inorganic compounds with excellent electrochemical properties and mechanical integrity to improve supercapacitor energy density at a new interval. This review presents new synthesis strategies to prevent the self-accumulation of MXene layers. First, the fundamental working theories of different supercapacitors are outlined. Next, an overview of the electrode material based on MXenes is outlined, and the latest solutions for increasing the active sites and improving the ion transfer rate have been collected. Hybridization and doping of MXenes change the properties of the composite, leading to a transformation in the structure and an increase in the capacitance. Furthermore, the utilization of double-transition metal MXenes solves challenges such as structural destruction and short life spans in multiple charge-discharge cycles. Then evaluation of the new MXene-based electrode materials in all-solid-state supercapacitors has been summarized. Finally, an overview of the latest developments in the creation of all-solid-state flexible supercapacitors as well as our predictions for future lines of inquiry is provided.
Downloads
References
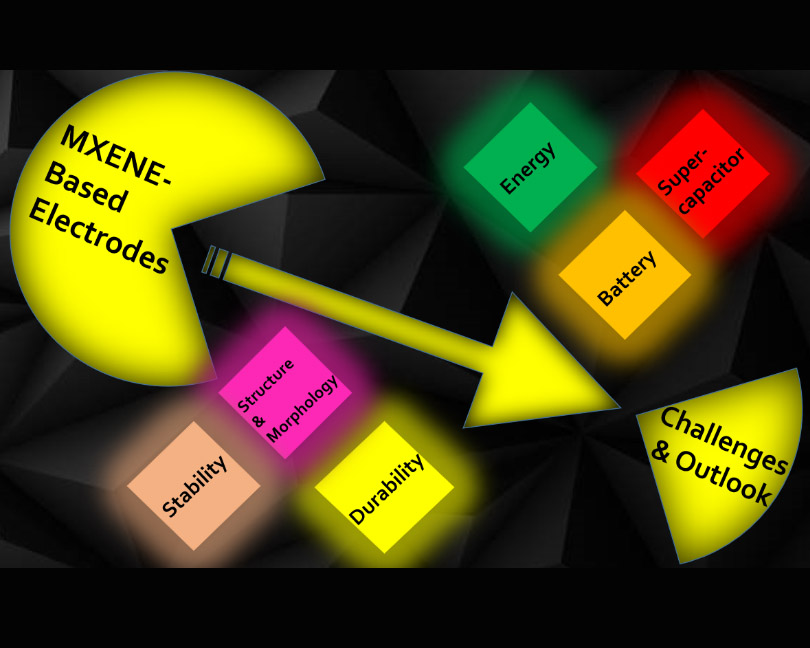
Copyright (c) 2023 Asieh Akhoondi, Mostafa Y. Nassar, Brian Yuliarto, Hicham Meskher

This work is licensed under a Creative Commons Attribution 4.0 International License.
Copyright
Authors are the copyright holders of their published papers in Synthesis and Sintering, which are simultaneously licensed under a Creative Commons Attribution 4.0 International License. The full details of the license are available at https://creativecommons.org/licenses/by/4.0/.
All papers published open access will be immediately and permanently free for everyone to read, download, copy, distribute, print, search, link to the full-text of papers, crawl them for indexing, pass them as data to software, or use them for any other lawful purpose without any registration obstacles or subscription fees.