Advancements in dental implant technology: the impact of smart polymers utilized through 3D printing
- 1 Department of Material Science and Engineering, Shahid Bahonar University of Kerman, Kerman, Iran
- 2 Department of Prosthodontics, School of Dentistry, Islamic Azad University, Tehran Dental Branch, Tehran, Iran
- 3 Department of Materials Engineering, Karaj Branch, Islamic Azad University, Karaj, Iran
- 4 Faculty of Dentistry, Urmia University of Medical Sciences, Urmia, Iran
Abstract
The field of dental implantology has witnessed significant advancements in recent years, driven by innovations in materials science and manufacturing technologies. One such innovation that holds promise for revolutionizing dental implant generation is mixing smart polymers through three-D printing. This evaluation article affords a comprehensive overview of the effect of clever polymers in enhancing the performance and functionality of dental implants. We begin by elucidating smart polymers' fundamental residences, which include their stimuli-responsive conduct, biocompatibility, and mechanical strength. Sooner or later, we discover the evolution and programs of 3D printing, e.g. direct metallic laser sintering (DMLS) and selective laser melting (SLM), in dentistry, highlighting its position in fabricating custom-designed dental implants. Combining smart polymers into dental implants is discussed in element, overlaying surface modification techniques, incorporation of bioactive dealers, and customization for affected person-particular desires. Furthermore, we look at how smart polymers make contributions to enhancing aspects such as osseointegration, peri-implantitis management, and average implant toughness. Clinical insights and case studies are presented to illustrate the real-global applications and results of clever polymer-based dental implants. Ultimately, this evaluation objective is to offer valuable insights for clinicians, researchers, and industry specialists worried about the improvement and utilization of advanced dental implant technologies.
Downloads
References
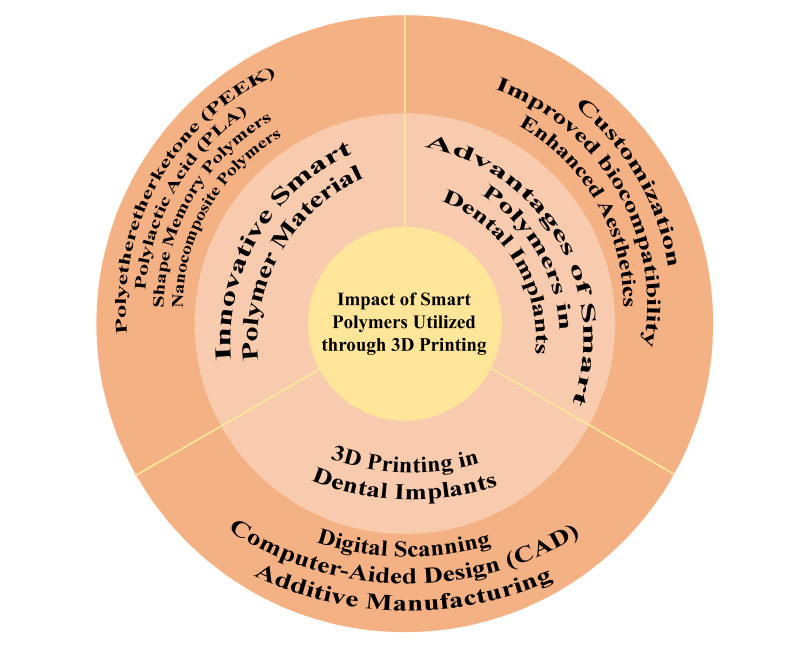
Copyright (c) 2024 Aliasghar Abouchenari, Neda Tajbakhsh, Amirhosein Shahbaz, Ghazal Alamdari-Mahd

This work is licensed under a Creative Commons Attribution 4.0 International License.
Copyright
Authors are the copyright holders of their published papers in Synthesis and Sintering, which are simultaneously licensed under a Creative Commons Attribution 4.0 International License. The full details of the license are available at https://creativecommons.org/licenses/by/4.0/.
All papers published open access will be immediately and permanently free for everyone to read, download, copy, distribute, print, search, link to the full-text of papers, crawl them for indexing, pass them as data to software, or use them for any other lawful purpose without any registration obstacles or subscription fees.