Effects of die geometry and insulation on the energy and electrical parameters analyses of spark plasma sintered TiC ceramics
- 1 Department of Mechanical Engineering, Faculty of Manufacturing, University of Tabriz, Tabriz, Iran
- 2 Department of Mechanical Engineering, University of Tarbiat Modares, Tehran, Iran
- 3 Department of Electrical Engineering, University of Mohaghegh Ardabili, Ardabil, Iran
Abstract
This work conducts a numerical simulation to investigate the temperature and electric current distribution during the spark plasma sintering (SPS) process using the finite element method (FEM) carried out in COMSOL Multiphysics software. The main goal is to optimize the SPS process for titanium carbide (TiC) ceramics, with a particular focus on the effects of insulation and die geometry (height and thickness). For the TiC material, the ideal sintering temperature is set at 2000 °C. The study analyzes eight case studies, involving a base case, an insulating case, and six cases with various thicknesses and heights, to evaluate the effectiveness of the suggested optimization. The results show that using insulation on the die surface reduces heat transfer from the die surface significantly, which leads to a 63% decrease in input power consumption when compared to the basic scenario. Based on a correlation study between energy and electricity, increasing die thickness raises the cross-sectional area of the electric current, which raises the amount of electric power required to attain the 2000 °C sintering temperature. The results indicate the temperature distribution in the sample is more sensitive to changes in die height than to changes in die thickness.
Downloads
References
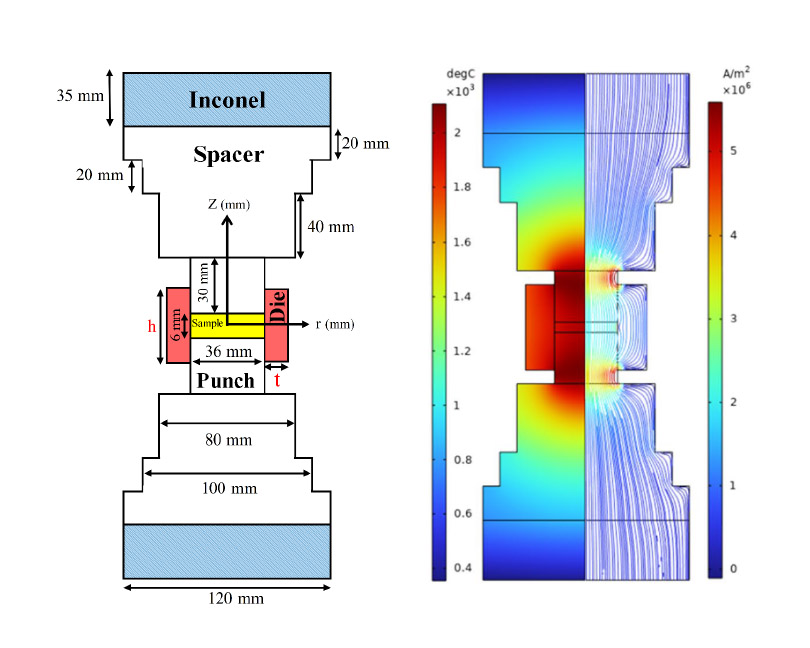
Copyright (c) 2024 Milad Sakkaki, Milad Foroutani, Peyman Zare

This work is licensed under a Creative Commons Attribution 4.0 International License.
Copyright
Authors are the copyright holders of their published papers in Synthesis and Sintering, which are simultaneously licensed under a Creative Commons Attribution 4.0 International License. The full details of the license are available at https://creativecommons.org/licenses/by/4.0/.
All papers published open access will be immediately and permanently free for everyone to read, download, copy, distribute, print, search, link to the full-text of papers, crawl them for indexing, pass them as data to software, or use them for any other lawful purpose without any registration obstacles or subscription fees.