A TEM study of nanostructures and interfaces in the hot-press sintered ZrB2–SiC–Si3N4 composites
- 1 Department of Metallurgy, Empress Catherine II Saint Petersburg Mining University, Saint Petersburg, Russia
- 2 Department of Petroleum Engineering, Empress Catherine II Saint Petersburg Mining University, Saint Petersburg, Russia
- 3 Independent Researcher, Quito, Ecuador
- 4 Department of Petroleum Engineering, China University of Petroleum-Beijing 18, Fuxue Road, Changping District, Beijing 102249, China
Abstract
A fully dense ZrB2–30 vol% SiC composite containing 5 wt% Si3N4 and 4 wt% phenolic resin (1.6 wt% carbon) was sintered using the hot-pressing route under the external pressure of 10 MPa at 1900 ºC for 2 h. The microstructural evolution and interfacial phenomena were scrutinized using advanced microscopy facilities such as high-resolution transmission electron microscopy (HRTEM) and field emission scanning electron microscopy (FESEM). The FESEM images showed the ZrB2 and SiC grains without any evidence of Si3N4. The formation of the hexagonal BN (hBN) phase was proven in the sintered composite. The hBN nanosheets had a graphite-like morphology with an average thickness of 20 nm. This phase has a perpendicular orientation to the pressure direction and prevents abnormal ZrB2 grain growth. Two types of ZrB2/SiC interfaces were detected, which exhibited an amorphous phase along with the grain boundary and a clean/smooth interface, resulting from the Si3N4 addition. HRTEM and inverse fast Fourier transform (IFFT) observations disclosed that the d-spacing value in the ZrB2 grain (0.335 nm) is higher than those reported in the literature. Furthermore, it was found that the exerted pressure during the sintering distorted atomic planes. The presence of numerous dislocations within the ZrB2 grains confirmed dislocation creep as the main densification mechanism.
Downloads
References
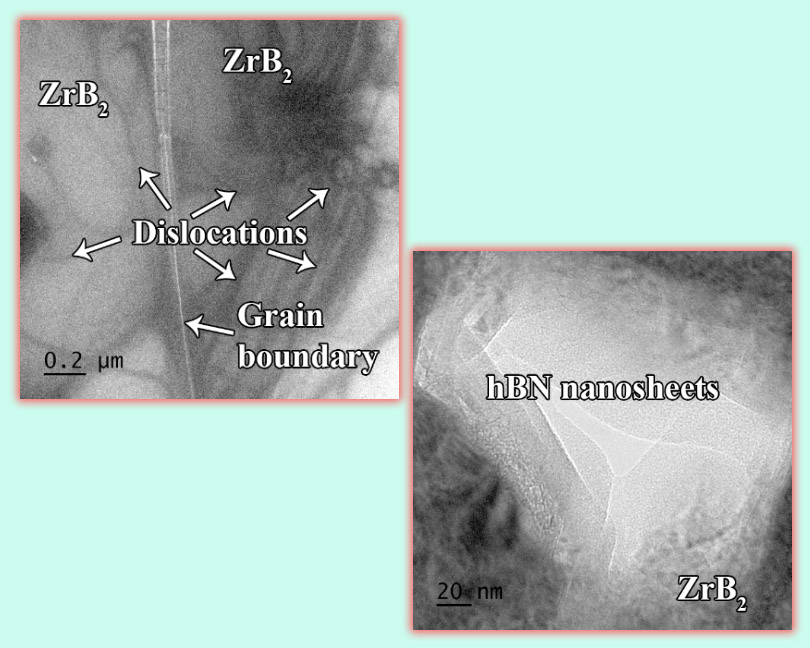
Copyright (c) 2023 Vladimir Bazhin, Aleksander Nikolaev, Valeria Esthefania Quiroz Cabascango, Changjin Shao, Genrih Davletov, Tatyana Gizatullina, Vadim Fetisov

This work is licensed under a Creative Commons Attribution 4.0 International License.
Copyright
Authors are the copyright holders of their published papers in Synthesis and Sintering, which are simultaneously licensed under a Creative Commons Attribution 4.0 International License. The full details of the license are available at https://creativecommons.org/licenses/by/4.0/.
All papers published open access will be immediately and permanently free for everyone to read, download, copy, distribute, print, search, link to the full-text of papers, crawl them for indexing, pass them as data to software, or use them for any other lawful purpose without any registration obstacles or subscription fees.