Synthesis and sintering of SrTiO3–ZnO ceramics: Role of ZnO content on microstructure and dielectric properties
- 1 Department of Metallurgical and Materials Engineering, University of Engineering and Technology, G.T Road, Lahore – 54890, Pakistan
- 2 NUS Graduate School for Integrative Sciences and Engineering, National University of Singapore, Singapore 119077, Singapore
- 3 Department of Metallurgical and Materials Engineering, University of Punjab Lahore, Pakistan
Abstract
The classical system of combining modern perovskite and wurtzite structure semiconductor materials is used to model the internal structure for the applications of functional novel electronic devices. The structure-property relation has a significant impact on the properties of metal oxides-based functional ceramics. The structural and electrical properties of SrTiO3-xZnO (0 ≤x≤ 10 wt%) ceramics produced via solid-state reaction (SSR) were thoroughly examined. X-ray diffraction (XRD) and scanning electron microscopy confirmed the presence of a mono-phase cubic structure with Pm3̅m space group and resulted in increased density respectively. Complex impedance spectroscopy (CIS) was carried out from 300 to 500 °C temperature within the frequency range of 100 Hz to 1 MHz to study the contribution of grain bulk and grain boundary for impedance behavior. Grain boundaries dominated the overall resistance of the samples and the addition of ZnO in SrTiO3 caused an increase in the overall conductivity. Increasing temperature decreases the resistance of both components, and at higher frequencies that confirms the negative temperature coefficient resistance (NTCR) behavior of the samples. Increasing temperature decreases the relaxation of grain bulk and grain boundary thus predicting the hopping conduction mechanism. The results will be helpful to engineer the microstructure of SrTiO3 based on practical applications such as sensors, actuators, and energy devices.
Downloads
References
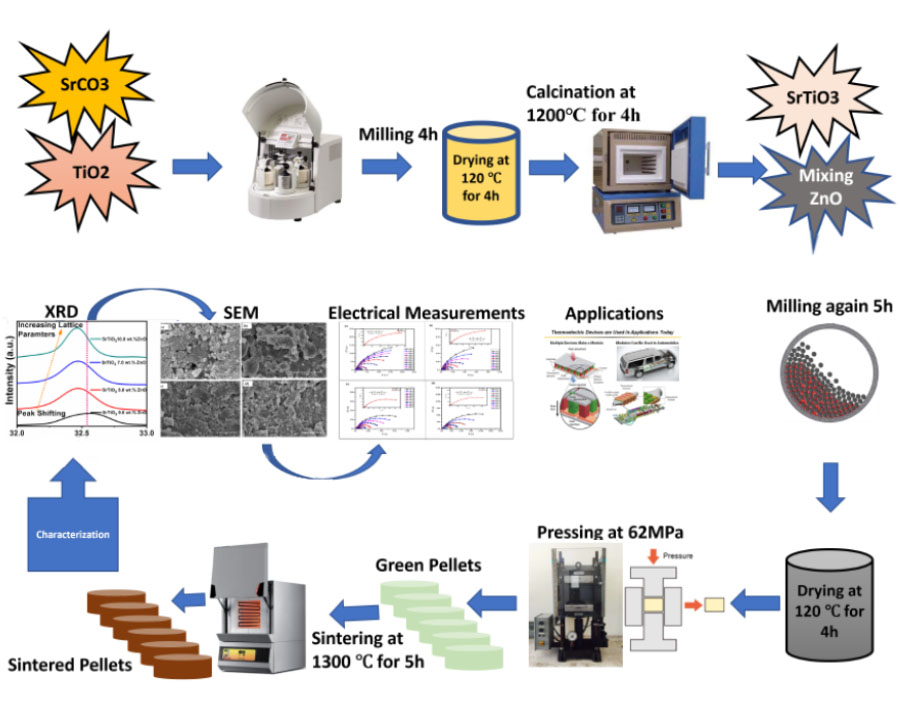
Copyright (c) 2023 Muhammad Kashif, Muhammad Salman Habib, Muhammad Asif Rafiq, Moaz Waqar, Muhammad Asif Hussain, Ayesha Iqbal, Mehboob Ahmed Abbasi, Shahid Saeed

This work is licensed under a Creative Commons Attribution 4.0 International License.
Copyright
Authors are the copyright holders of their published papers in Synthesis and Sintering, which are simultaneously licensed under a Creative Commons Attribution 4.0 International License. The full details of the license are available at https://creativecommons.org/licenses/by/4.0/.
All papers published open access will be immediately and permanently free for everyone to read, download, copy, distribute, print, search, link to the full-text of papers, crawl them for indexing, pass them as data to software, or use them for any other lawful purpose without any registration obstacles or subscription fees.